ISSN: 0973-7510
E-ISSN: 2581-690X
Marine ecosystems house a plethora of microorganisms capable of producing therapeutically important metabolites, but remains largely unexplored. The aim of this work was to find a new marine bacterium capable of producing a fibrinolytic enzyme, which is fibrin specific, stable at the physiological pH, exhibiting both anticoagulant and thrombolytic effect in vitro. Once a potential bacterium was found, an effort was made to optimize medium. Based on the screening studies, the isolate C7 was chosen for further studies and was identified as Serratia marcescens subsp. sakuensis. Medium optimisation using Plackett-Burman design and one-factor experiments suggested that nitrogen sources have a profound effect on enzyme production. Fibrinolytic enzyme activity increased to 2.5 fold (487.29 U/mL) in the optimised medium as compared to unoptimised medium (191.65 U/mL). Current study demonstrates that seawater could be an excellent source for novel marine organisms capable of producing fibrinolytic enzymes. Fibrinolytic enzyme produced by Serratia marcescens subsp. sakuensis appears to be a potential candidate for thrombolytic therapy due to its high specific fibrinolytic activity, high ratio of fibrinolytic to fibrinogenolytic activity, activity at the physiological pH, anticoagulant and thrombolytic effects.
Anticoagulant; fibrinogenolytic, fibrinolytic, marine, thrombolytic.
Fibrinolytic enzymes carry out fibrinolysis by breaking down fibrin clot. Under normal physiological conditions of the human body, fibrin mesh is sliced by an enzyme plasmin (EC 3.4.21.7), releasing various fibrin degradation products1,2. Plasmin circulates in the form of inactive plasminogen, in turn activated by the tissue plasminogen activator (tPA) and urokinase upon injury. The understanding of in vivo lysis of fibrin clots has helped in developing enzyme based therapy2. The in vivo lysis of fibrin clots occurs by two ways, one being the direct lysis and the other being the indirect lysis. The former involves the action of plasmin like enzymes, which act readily on the fibrin clot, while the latter involves the action of enzymes that convert inactive plasminogen to active plasmin, which in turn degrade the fibrin clot. Hence, these clot-busting enzymes find applications in coronary thrombi leading to atrial fibrillation and myocardial infarctions, cerebral thrombi leading to strokes, pulmonary thromboemboli leading to cardiac and respiratory failure2.
Typically, a thrombus (blood clot) comprises of aggregated platelets (that form the plug) and a mesh of cross-linked fibrin proteins, the final product of the blood coagulation cascade. Often, thrombolytic agents are administered for acute myocardial infarction, wherein, the drugs act on the pre-existing thrombus or the whole clot, either by enhancing body’s own fibrinolytic pathways or by mimicking certain natural thrombolytic agents such as tissue plasminogen activator (tPA). The fibrinolytic agents available today for clinical use are urokinase, tPA and streptokinase, a bacterial plasminogen activator3. Certain recombinant tPA such as Alteplase, Retaplase and Tenectaplase, and Anisterplase, which is a combination of streptokinase and tPA is also drawing much attention4. However, these are tagged with limitations such as severe bleeding complications, lower fibrin specificity, short half-lives, larger therapeutic dose, allergic responses, and lysis of normal haemostatic plugs, in addition to their high costs4,5. These limitations advocate the exploration for novel fibrinolytic enzymes from newer microbial sources. Attempts have been made to discover fibrinolytic enzyme producing microbes from microorganism from edible mushroom6, fermented foods7 and specialised niches8.
Marine ecosystem houses a plethora of microorganisms, which till date remains largely unexplored. Owing to their habitat, these marine microbes are salt tolerant, possess a diverse range of enzyme activities, and specialized mechanisms to adapt to the extreme conditions9. There have been a few reports stating the anticoagulant nature of the compounds derived from marine algae10, Bacillus subtilis11,12,13 and also micromycetes14. Taking into account the saline nature of the seawater, the therapeutic enzymes of this origin are believed to provide an environment similar to that of the human blood, hence could be safer to use with less or no toxicity15.
Considering the significance of microorganisms of marine origin, as a source for the production of therapeutic enzymes, the present work was aimed at obtaining fibrinolytic enzymes that are fibrin specific, showing a significant fibrinolytic activity, while being safe for human use. Hence, experiments were designed to employ screening strategies to select a marine microorganism capable of producing a fibrinolytic enzyme, which is relatively more specific to fibrin than fibrinogen, stable at the physiological pH, exhibiting both anticoagulant and thrombolytic effect in vitro. Once the organism is selected based on screening studies, genotypic characterization was carried out to identify the selected one. Further attempts were made to enhance enzyme activity by medium optimisation.
Fibrin, thrombin, fibrinogen (all of human plasma origin), bovine serum albumin powder and sephadex G-100 were purchased from Sigma-Aldrich, India. Folin & Ciocalteu’s phenol reagent and trichloroacetic acid were sourced from LOBA Chemie, India. Starch casein agar, agar, asparagine, Luria Bertani broth, dextrose, maltose, D-(+)-galactose, soya peptone, yeast extract powder and dialysis membranes-150 were purchased from HiMedia laboratories, India. Anhydrous dextrose was obtained from Merck, India. NaCl, K2HPO4, MgSO4·H2O, CaCO3, CaCl2·2H2O, FeSO4 were purchased from Spectrum, Nice chemicals, India. All other chemicals and reagents used were of analytical grade.
Isolation and maintenance of microorganisms
Sea water collected from a depth of 10m, 5km away from Surathkal Coast in the Arabian Sea, was used for the isolation of microorganisms. Sea water samples were serially diluted (up to 10-7) and were plated on both starch casein agar and glucose asparagine agar and incubated at 37°C for 48 h Composition of the glucose asparagine agar was (g/L): glucose, 10; asparagine, 0.5; K2HPO4, 0.5; agar, 15 and pH 7. Individual colonies after 48h of incubation were picked and transferred onto starch casein agar plates having antifungal agent, amphotericin (50 µg/mL). Cultures were lyophilised using a combination of 10% (w/v) sucrose and 10% (w/v) skimmed milk as cryoprotectants and stored in refrigerator for long-term use.
Qualitative screening
The fibrinolytic activity of the isolates was screened using the method described previously16 with minor modifications. The cultures were plated on modified fibrin agar screening medium with a composition of 1.2% (w/v) agarose solution, 40 mg/mL human fibrinogen solution (prepared in 0.9% w/v saline) and 100 U/mL human thrombin solution. These plates were then incubated at 37°C for 7 days. The appearance of a turbid zone initially, followed by the appearance of clear zone within the turbid zone indicated the production of fibrinolytic enzyme.
The isolates, which showed turbid and clear zones, were inoculated into a sterile unoptimised medium having composition (g/L): glucose, 20; peptone, 10; yeast extract, 10 ; KH2PO4·3H2O, 1; MgSO4· 7H2O, 0.5; CaCl2·2H2O, 0.5 g/L and pH 7, incubated at 37°C for 48 h. The cell free supernatant obtained by centrifuging the fermentation broth at 12,000 × g at 4 ºC for 10 min, was taken to confirm the presence fibrinogenolytic and fibrinolytic activities according to the method described by Shivaprasad et al.[17] with minor modifications. The cell free broth was mixed with an equal volume of fibrinogen solution (prepared by dissolving 10 mg in 50 mL of 0.9% w/v NaCl solution). Absorbance of the mixture was read at 540 nm in UV/Vis spectrophotometer (LabIndia) every 15 min, starting from 0th min to 210th min against a suitable blank. The experiments were carried out in duplicates and the mean values were plotted. Thrombin (100 U/mL) was used as the control.
Assay for fibrinolytic activity
To estimate fibrinolytic activity, the method described by Agrebi et al.11 was used with fibrin as a substrate. Substrate solution was prepared by mixing 10 mg of fibrin in 100 mL of 0.1 M glycine-NaOH buffer (pH 9). One millilitre enzyme solution was mixed with equal volume of substrate solution, incubated at 55 °C for 15 min, 0.2M TCA solution (1 mL) was added and incubated at room temperature for 15 min to stop the reaction. The contents were centrifuged at 10,000 × g for 15 min at 4°C to remove the precipitate. Absorbance of the supernatant was read at 280 nm against a suitable blank. A standard graph was constructed using different concentrations of tyrosine, ranging between 0 – 100 µg/mL and the absorbance was read at 280 nm. One unit of fibrinolytic enzyme activity was expressed as 1 µg of tyrosine liberated per min at 55°C.
Assay for fibrinogenolytic activity
The fibrinogenolytic assay was carried out as described by Wang et al.[18] with slight modifications. In brief, substrate solution was prepared by mixing 10mg of fibrinogen in 50 mL of 0.9% (w/v) NaCl solution. One milliliter of the substrate solution was mixed with 0.5 mL of 245 mM phosphate buffer (pH 7) and incubated at 37°C for 5 min. As our aim was to estimate the fibrinogenolytic activity, addition of thrombin was avoided, since thrombin converts fibrinogen to fibrin. One millilitre of the diluted enzyme solution was added to the reaction mixture, followed by mixing for 5 min at 30°C. The reaction was stopped by adding 1 mL of 0.2M TCA solution. This reaction mixture was centrifuged at 10,000 × g for 10 min at 4 °C and the absorbance of the supernatant was read at 280 nm. One unit of fibrinogenolytic enzyme activity is expressed as 0.01 per min increase in absorbance at 280 nm.
Anticoagulant activity
In vitro anticoagulant activity of the fibrinolytic enzymes was examined by the method described by Lu et al.19 with minor modifications. This work was carried out upon receiving the approval from the ethical committee and obtaining an informed consent from the human volunteers (healthy male volunteers) for the experimentation. One millilitre of fresh human whole blood was added to equal volume of cell free crude enzyme solutions (suitably diluted with 0.9 % w/v NaCl, pH 7.4), in a sterile glass test tube, mixed gently and incubated at 37 ºC for 6 h. Filter sterilised saline (0.9 % w/v NaCl, pH 7.4) was used as negative control. Coagulation of the blood was monitored with respect to control. Upon incubation for 6 h the supernatant liquid was decanted and the extent of clot formed was observed visually.
Thrombolytic activity
The thrombolytic effect of the fibrinolytic enzymes was assessed according to the method described by Lu et al.19 with minor modifications. This work was carried out upon receiving the approval from the ethical committee and obtaining an informed consent from the human volunteers (healthy male volunteers) for the experimentation. Briefly, one milliliter of fresh human whole blood was allowed to clot upon incubation at 37ºC for 1h. Subsequently, to the solid clot formed, 1mL of cell free crude enzyme solutions (suitably diluted with 0.9 % w/v NaCl, pH 7.4) were added and incubated at 37 ºC for 6 h. Filter sterilised saline (0.9 % w/v NaCl, pH 7.4) was used as negative control. Upon incubation for 6h the supernatant liquid was decanted and the extent of clot lysed was observed visually.
Partial purification of the fibrinolytic enzyme
Four isolates selected based on the initial screening studies were cultured in the previously mentioned medium. The cell free crude enzyme solutions of cultures C1, C3, C5 and C7 were then subjected to ammonium sulphate salt precipitation at ice cold conditions. Initial trials were carried out, with varying ammonium sulphate concentrations (40%, 50%, 60%, 70%, and 80% saturation (w/v)), in order to determine an optimal percentage saturation of the salt required for precipitation of fibrinolytic enzyme. Care was taken to ensure that the desired final concentration of ammonium sulphate was achieved by gradually adding increasing concentrations of the salt at each step, following which the samples were centrifuged. The precipitated proteins were later dialysed against 20 mM sodium phosphate buffer of pH 7.
Protein concentration
Protein concentration of cell free broth and enzyme solutions was determined using a method as described previously[20], using BSA (Bovine Serum Albumin) as standard and measuring the absorbance at 660 nm.
Effect of pH on the partially purified enzyme
The dialysed protein solutions were incubated with the respective buffers ranging from pH 4.0 to 9.0 for 30 min at room temperature and residual fibrinolytic enzyme activities were determined. All experiments were carried in duplicates and the average values were calculated.
Identification of the isolate
Out of all the isolates obtained, only one microorganism was chosen based on aforesaid mentioned criteria and was characterised by 16S rRNA gene sequencing and the phylogenetic analysis was carried out (by Microbial Culture Collection, National Centre for Cell Science, Pune, India), the report was generated using EZ Taxon Database.
Medium optimisation
In order to determine the factors having a major effect on the fibrinolytic enzyme production the Plackett-Burman design matrix was constructed using Stat-Ease Design Expert software v7.0.0 (Table 1). A total of nineteen (n) variables comprising nutritional factors such as concentration of dextrose, galactose, maltose, yeast extract, soya peptone, NaCl, K2HPO4, MgSO4, CaCO3, FeSO4, sea water (passed through 0.22 µm filter) and eight dummy variables were screened in twenty (n+1) experiments. Each variable was examined at low (-1) and high (+1) level of concentrations. The concentrations of the factors being dextrose (1-4% w/v), galactose (1- 4% w/v), maltose (1 – 4% w/v), yeast extract (0.5 – 2% w/v), soya peptone (0.5 – 2% w/v), NaCl (0.01 – 0.05% w/v), K2HPO4 (0.01 – 0.05% w/v), MgSO4 (0.05 – 0.15% w/v), CaCO3 (0.05 – 0.15% w/v), FeSO4 (0.01 – 0.05% w/v), sea water (1 – 5% v/v). All the trials were conducted in 250mL Erlenmeyer flasks, with a medium volume of 100 mL, with 5 % (v/v) inoculum concentration. The initial medium pH was 7.0 to 7.2, incubated in an incubator shaker at 150rpm, at 37ºC for 48h. Samples were withdrawn after every 8h. All experiments were carried out in duplicates, the average of enzyme activities were calculated and taken as response. The data obtained was analysed with the help of Design Expert v7.0.0. Pareto chart and normal plot were constructed to determine the most significant factors that influence the fibrinolytic enzyme production.
Table (1):
Plackett-Burman design matrix generated by Design Expert 7.0.
Std | Run | Block | Factors* | Response 1Total Enzyme Activity U/mL |
||||||||||
---|---|---|---|---|---|---|---|---|---|---|---|---|---|---|
1 A | 2 B |
3 C |
4 D |
5 E |
6 F |
7 G |
8 H |
9 J |
10 K |
11 L |
||||
1 | 1 | Block 1 | 1 | 1 | -1 | -1 | 1 | 1 | 1 | 1 | -1 | 1 | -1 | 290.27 |
16 | 2 | Block 1 | 1 | 1 | 1 | 1 | -1 | 1 | -1 | 1 | -1 | -1 | -1 | 257.47 |
3 | 3 | Block 1 | 1 | -1 | 1 | 1 | -1 | -1 | 1 | 1 | 1 | 1 | -1 | 264.85 |
5 | 4 | Block 1 | -1 | 1 | 1 | -1 | 1 | 1 | -1 | -1 | 1 | 1 | 1 | 261.8 |
15 | 5 | Block 1 | 1 | 1 | 1 | -1 | 1 | -1 | 1 | -1 | -1 | -1 | -1 | 296.63 |
13 | 6 | Block 1 | 1 | -1 | 1 | -1 | 1 | -1 | -1 | -1 | -1 | 1 | 1 | 317.99 |
7 | 7 | Block 1 | -1 | -1 | -1 | 1 | 1 | -1 | 1 | 1 | -1 | -1 | 1 | 259.26 |
9 | 8 | Block 1 | 1 | -1 | -1 | -1 | -1 | 1 | 1 | -1 | 1 | 1 | -1 | 289.25 |
11 | 9 | Block 1 | 1 | -1 | 1 | -1 | -1 | -1 | -1 | 1 | 1 | -1 | 1 | 288.75 |
19 | 10 | Block 1 | 1 | -1 | -1 | 1 | 1 | 1 | 1 | -1 | 1 | -1 | 1 | 265.61 |
2 | 11 | Block 1 | -1 | 1 | 1 | -1 | -1 | 1 | 1 | 1 | 1 | -1 | 1 | 247.05 |
17 | 12 | Block 1 | -1 | 1 | 1 | 1 | 1 | -1 | 1 | -1 | 1 | -1 | -1 | 239.42 |
6 | 13 | Block 1 | -1 | -1 | 1 | 1 | -1 | 1 | 1 | -1 | -1 | 1 | 1 | 269.93 |
20 | 14 | Block 1 | -1 | -1 | -1 | -1 | -1 | -1 | -1 | -1 | -1 | -1 | -1 | 261.55 |
14 | 15 | Block 1 | 1 | 1 | -1 | 1 | -1 | 1 | -1 | -1 | -1 | -1 | 1 | 278.32 |
4 | 16 | Block 1 | 1 | 1 | -1 | 1 | 1 | -1 | -1 | 1 | 1 | 1 | 1 | 309.59 |
12 | 17 | Block 1 | -1 | 1 | -1 | 1 | -1 | -1 | -1 | -1 | 1 | 1 | -1 | 342.14 |
18 | 18 | Block 1 | -1 | -1 | 1 | 1 | 1 | 1 | -1 | 1 | -1 | 1 | -1 | 405.95 |
10 | 19 | Block 1 | -1 | 1 | -1 | -1 | -1 | -1 | 1 | 1 | -1 | 1 | 1 | 376.97 |
8 | 20 | Block 1 | -1 | -1 | -1 | -1 | 1 | 1 | -1 | 1 | 1 | -1 | -1 | 346.72 |
*A:Dextrose g/L, B:Galactose g/L, C:Maltose g/L, D:Yeast Extract g/L, E:Soya Peptone g/L, F:NaCl g/L,G:K2HPO4 g/L, H:MgSO4 g/L, J:CaCO3 g/L, K:FeSO4 g/L, L:Sea Water mL/L
Experiments were conducted to ascertain the effect of carbon sources on the fibrinolytic enzyme production, when incorporated into the medium. A flask with no carbon source having the following components (% w/v): yeast extract 2, soya peptone 2, NaCl 0.05, CaCO3 0.15, FeSO4 0.05 and seawater 5% v/v was used as control. Different carbon sources such as dextrose, galactose, maltose and starch, with their respective concentrations maintained constant at 1% w/v were added one at a time, while the other components remained the same as that in control.
Based upon the results obtained from Plackett-Burman design of experiments, a separate set of experiments were conducted to determine the effect of various nitrogen sources, which were found to be highly significant. The concentration of nitrogen sources were varied in the range of 3% w/v to 9% w/v. While the other components with their respective concentrations were their respective concentrations were (% w/v): soya peptone 2, NaCl 0.05, CaCO3 0.15 and FeSO4 0.05.
Isolation and screening
In this study, eight bacterial strains designated as C1, C2, C3, C4, C5, C6, C7 and C8 were isolated from the seawater sample and in the initial screening experiments using modified fibrin agar plates, six isolates tested positive for fibrinogenolytic and fibrinolytic enzyme production. The appearance of turbid zone during the initial 48h of incubation, followed by the appearance of clear zone within the turbid zone after prolonged incubation for 7 days (Figure 1A) indicated the presence of extracellular enzyme. Colony morphologies of the 6 isolates that tested positive for the production of fibrinogenolytic/fibrinolytic enzyme were studied (Table 2).
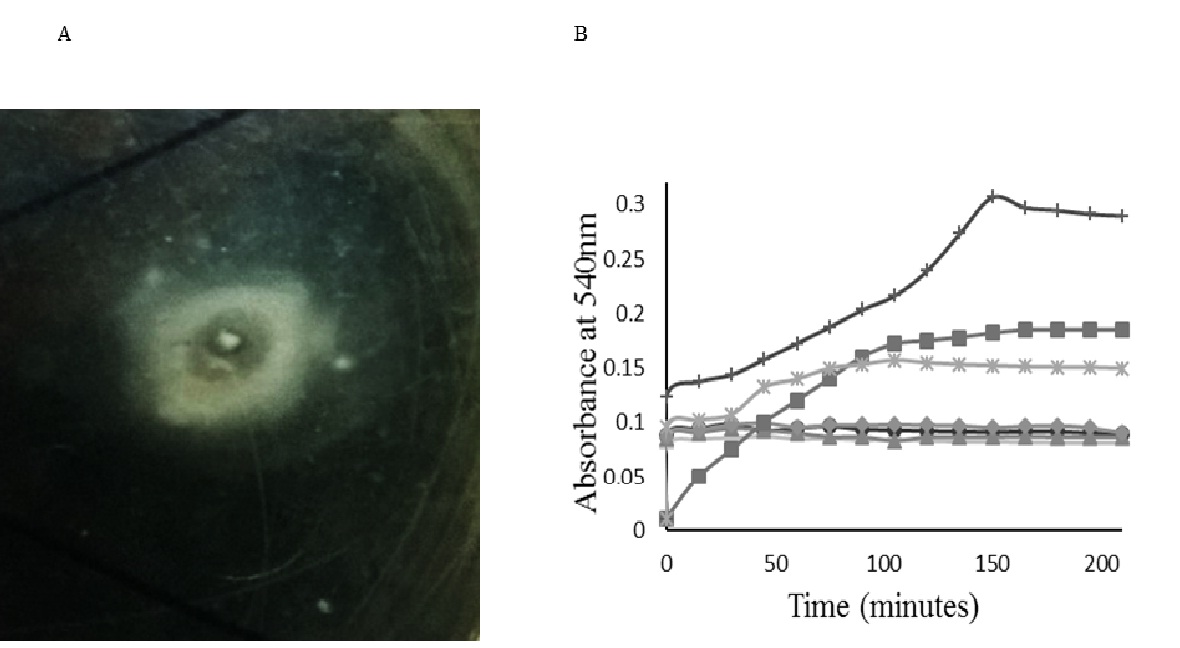
Figure 1 Screening A) Qualitative screening (appearance of clear zone within the turbid zone on modified fibrin agar plate), B) Spectrophotometric analysis for the confirmation of presence of the fibrinogenolytic and fibrinolytic activities of the cell free crude enzyme solutions: C1 (closed diamond), C3 (closed circle), C5 (minus), C6 (plus), C7 (closed triangle), C8 (cross). Absorbance of the substrate incubated with thrombin (closed square) was also recorded, and used as control. Experiments were conducted in duplicates, and results were plotted as mean
Table (2):
Colony morphologies and gram staining properties of the six isolates.
Isolates |
Colour |
Shape |
Elevation |
Margin |
Surface |
Density |
Consistency |
Gram stain |
---|---|---|---|---|---|---|---|---|
C1 |
White |
Filamentous |
Flat |
Filamentous |
Smooth |
Translucent |
Hard to get off |
+ve |
C3 |
Grey |
Irregular |
Raised |
Undulate |
Rough |
Opaque |
Dry |
+ve |
C5 |
White |
Spindle, filamentous |
Flat |
Entire |
Smooth |
Transparent |
Hard to get off |
+ve |
C6 |
Orange |
Circular |
Convex |
Entire |
Glistening |
Opaque |
Mucoid |
+ve |
C7 |
White |
Filamentous |
Convex |
Entire |
Smooth |
Translucent |
Viscid |
-ve |
C8 |
Creamish white |
Circular |
Convex |
Entire |
Smooth |
Opaque |
Butyrous |
+ve |
Qualitative measurement of fibrinolytic/fibrinogenolytic activity of cell free broth showed that all the six isolates showed instant increase in the absorbance when cell free broth was mixed with fibrinogen solution (Figure 1B). On the contrary, thrombin solution, which was used as the control, took almost 50 min to show the same degree of absorbance. In case of isolates C8 and C6, the absorbance showed an increasing trend up to 105 min and 150 min respectively, whereas other isolates did not show any further increase in absorbance. However, the duration of both fibrinogenolytic and fibrinolytic activities observed varied among isolates.
As the qualitative screening could only confirm the presence of fibrinolytic/fibrinogenolytic activity of the selected isolates, accurate determination of fibrinolytic activity and fibrinogenolytic activity of each individual isolate was necessary to select the most desired one. Using specific estimation methods, fibrinogenolytic and fibrinolytic activities for the cell free crude enzyme extracts of all 6 isolates were determined, and the simplified ratios of fibrinolytic to fibrinogenolytic activity were calculated accordingly, as seen in Table 3. The protein concentration of cell free broth was determined for all the cultures and was used to calculate specific fibrinolytic and fibrinogenolytic activity of all the culture broths. The isolate C5 showed the highest specific fibrinolytic and fibrinogenolytic activity (Table. 3). However, the ratio of specific fibrinolytic to specific fibrinogenolytic activity was 7:1 for C5 isolate while, it was 8:1 for C7 isolate. This indicated that the enzyme produced is primarily a fibrinolytic enzyme and that fibrinogenolytic activity could be an additional function due to the availability of fibrinogen as substrate.
Table (3):
Fibrinolytic and fibrinogenolytic activities of the cell free crude enzyme solutions of the six isolates.
Isolate |
Total Protein (mg) |
Total Fibrinolytic Enzyme Activity (U/ml) |
Specific Fibrinolytic Enzyme Activity* (U/mg protein) |
Total Fibrinogenolytic Enzyme Activity (U/ml) |
Specific Fibrinogenolytic Enzyme Activity* (U/mg protein) |
Fibrinolytic: Fibrinogenolytic ratio |
---|---|---|---|---|---|---|
C7 |
2.51 |
191.65 |
76.26 ± 0.03 |
24.95 |
9.93 ± 0.01 |
8:1 |
C5 |
2.19 |
195.67 |
88.98 ± 0.07 |
27.93 |
12.70 ± 0.07 |
7:1 |
C3 |
2.31 |
181.28 |
78.44 ± 0.28 |
28.81 |
12.46 ± 0.02 |
7:1 |
C1 |
2.76 |
158.40 |
57.49 ± 0.01 |
27.89 |
10.12 ± 0.04 |
6:1 |
C6 |
2.47 |
163.25 |
66.01 ± 0.0 |
27.87 |
11.26 ± 0.01 |
6:1 |
C8 |
2.73 |
186.97 |
68.51 ± 0.10 |
27.18 |
9.95 ± 0.0 |
7:1 |
*Data represents mean ± SD (n = 2).
Anticoagulant activity
As shown in Figure 2A, clotting of blood had occurred in presence of normal saline (control) after 6h whereas, in presence of the crude enzyme solutions, the clot formation was reduced. Particularly, in the test tube containing crude enzyme extract produced by C7 isolate, a miniscule amount of blood clot was formed after 6h. These results indicated that the crude enzyme extracts exhibit significant anticoagulant activity in vitro as compared to the control.
Thrombolytic activity
Thrombolytic activity of the crude enzymes was indicated by the blood clot dissolution upon incubation for 6h (Figure 2B). However, in the control tubes (normal saline) there was no clot dissolution. The enzymes produced by the isolates C5 and C7 showed relatively greater clot lysis in comparison to the other enzymes. The results obtained indicated the thrombolytic action of the fibrinolytic enzymes produced.
Partial purification and effect of pH
Four isolates (C1, C3, C5 and C7) were selected based on the initial screening studies. Although C1 showed a relatively lesser fibrinolytic activity (Table 3), it showed reasonable clot lysis upon observation during in vitro trials (Figure 2). Further, the cell free supernatants of these isolates were partially purified and their fibrinolytic activities were estimated (Table 4). The effect of varying pH on the partially purified enzymes was also studied, in order to understand the optimum pH range for the enzyme (Figure 3). Isolate C7 showed a maximum specific activity of 104.89 U/mg at pH 6.0 and 80.58 U/mg at pH 7.0, showing about 23% decrease in activity. However, in comparison to other isolates, the maximum specific activity showed by C7 in the human physiological pH range is higher than other isolates. In addition, enzyme from C7 isolate also showed excellent anticoagulant and thrombolytic effect in vitro, and showed higher fibrinolytic / fibrinogenolytic activity ratio (8:1) compared to the enzymes from other isolates. Hence, C7 was chosen for further studies.
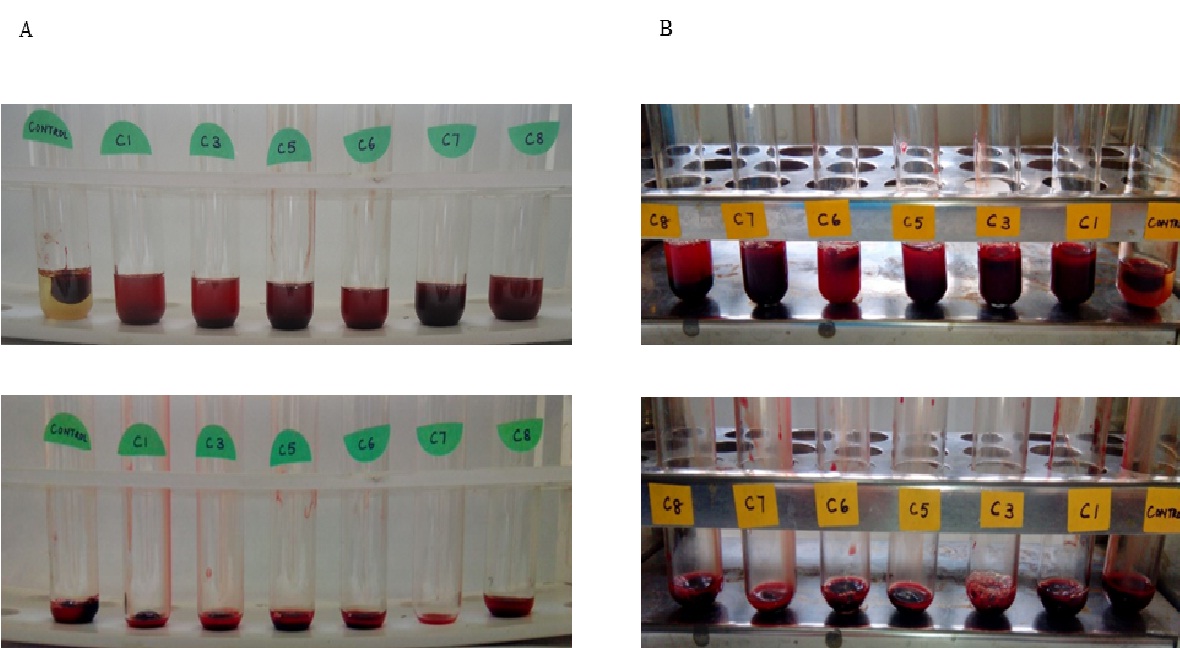
Figure 2 In vitro assays A) Visualisation of anticoagulant effect of the cell free crude enzyme solutions on human blood in vitro; formation of blood clots after incubation with the cell free crude enzyme solutions for 6 hours, the plasma decanted, B) Visualisation of lysis of pre-formed blood clots by the cell free crude enzyme solutions in vitro; clot lysis observed upon 6 hours of incubation of blood clots with the cell free crude enzyme solutions, the plasma decanted.
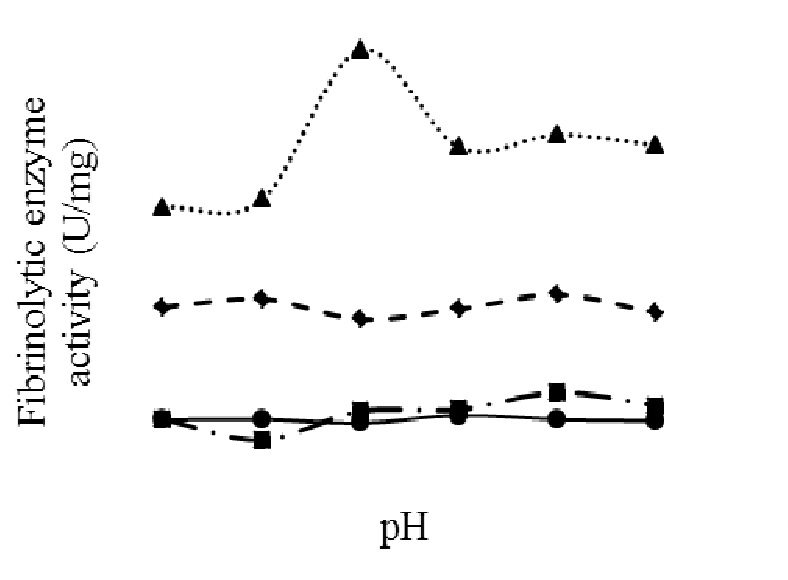
Figure 3 Effect of pH on fibrinolytic activity of partially purified fibrinolytic enzyme of the four isolates: C1 (closed circle), C3 (closed square), C5 (closed diamond), C7 (closed triangle)
Table (4):
Fibrinolytic activity upon partial purification.
SL No. |
Isolate |
Specific Fibrinolytic Enzyme Activity* (U/mg protein) |
---|---|---|
1 |
C1 |
10.91 ± 0.02 |
2 |
C3 |
24.46 ± 0.24 |
3 |
C5 |
64.02 ± 0.12 |
4 |
C7 |
90.30 ± 0.1 |
*Data represents mean ± SD (n = 2).
Identification of isolate C7
The organism was identified as Serratia marcescens subsp. sakuensis by 16S rRNA gene sequencing. The sequence data showed a pair wise sequence similarity of 99.72% with Serratia marcescens subsp. sakuensis21. The phylogenetic tree of C7 is shown in Figure 4, and the 16S rRNA sequence was submitted to GenBank database at NCBI with the accession number KU296189.1.
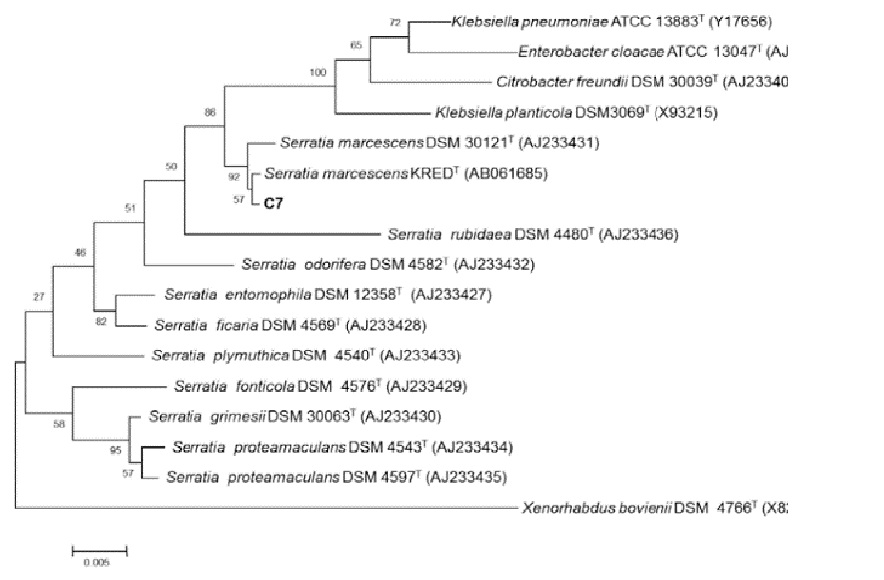
Figure 4 Phylogenetic tree of the isolate C7
Medium optimisation
Selection of the most significant factor was made using the Plackett-Burman design. The design matrix (Table 1) was generated by Design Expert 7.0 (Stat ease, Inc.). The mean square value and F test value for yeast extract (D) and soya peptone (E) was higher than that for other nutritional and dummy variables indicating that these two are important nutritional components affecting the fibrinolytic enzyme production (data not shown). Larger F value of 22.79 and p value of 0.0001 implied that the model was significant. The coefficient of determination, R2 for the model was 0.9691 showing the goodness of fit of the model. The adjusted R2 was 0.9265. These values indicate good adequacy of the model. The Pareto chart offers a convenient way to view the results obtained. It shows the significance of the variables affecting the enzyme production. From the Pareto chart (Figure 5) it can be observed that only two factors namely yeast extract and soya peptone out of eleven nutritional factors had a large positive influence on the fibrinolytic enzyme production. Nevertheless, galactose, FeSO4, sea water, CaCO3 and NaCl showed minimal positive effect on the enzyme production. None of the carbon sources incorporated in the study was found to have a major positive significant effect on the fibrinolytic enzyme production. Further experiments were performed to confirm the minimal significant contribution of carbon source when incorporated in the medium. From Table 5 it was observed that there was a marginal change in fibrinolytic enzyme activity as compared to that in the control where no carbon source was incorporated. The result obtained has showed that incorporation of carbon source does not enhance the fibrinolytic enzyme production significantly.
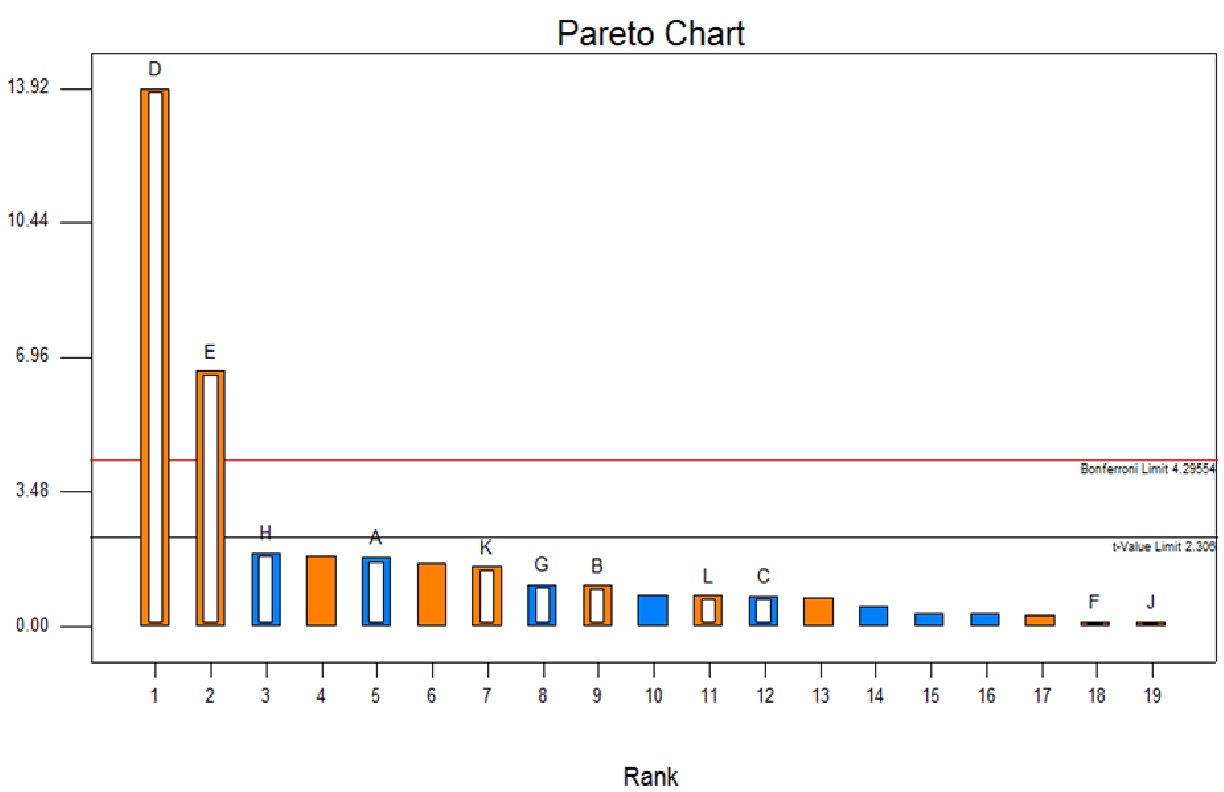
Figure 5 Effect of medium components using Plackett-Burman design A) Pareto chart (A-Dextrose, B-Galactose, C-Maltose, D-Yeast Extract, E-Soya Peptone, F- NaCl, G- K2HPO4, H- MgSO4, J- CaCO3, K- FeSO4, L-Sea water)
Table (5):
Effect of carbon source on fibrinolytic enzyme production.
SL No. |
Carbon Source |
Total Enzyme Activity* (U/mL) |
---|---|---|
1 |
None (Control) |
229 ± 3.28 |
2 |
Dextrose |
239.93 ± 0.71 |
3 |
Galactose |
231.79 ± 3.88 |
4 |
Maltose |
228.74 ± 1.98 |
5 |
Starch |
214.26 ± 4.24 |
*Data represents mean ± SD (n = 2).
As it was observed that the incorporation of nitrogen source contributed significantly to the production of fibrinolytic enzyme, separate set of experiments were conducted by varying the concentration of most Table 6 significant factor (yeast extract) (Figure 5) and the fibrinolytic enzyme activity was determined (Table 6). From the results obtained, it was observed that, with the increase in the concentration of yeast extract the enzyme activity also increased (487.29 U/mL). There was a 2.5 fold increase in the fibrinolytic enzyme activity compared to an enzyme activity of 191.65 U/mL obtained in an unoptimised medium (Table 3). The results suggest that the organism has a remarkably high nitrogen demand.
Table (6):
Effect of varying concentration of yeast extract on fibrinolytic enzyme production.
SL No. | Yeast Extract (% w/v) | Total enzyme activity* (U/mL) | |
---|---|---|---|
1 | 30 | 381.85 ± 0.73 | |
2 | 60 | 448.12 ± 0.45 | |
3 | 90 | 487.29 ± 1.00 |
*Data represents mean ± SD (n = 2).
Specificity of the fibrinolytic enzyme towards fibrin becomes a critical parameter while evaluating its potential as therapeutic agent. A quick review of the existing literature, showed that most of the microorganisms capable of producing a fibrinolytic enzyme, also produce fibrinogenolytic enzyme[22,23], whereby making the enzyme less fibrin specific. In these reports, time dependent degradation of fibrinogen by the enzyme has been demonstrated by loading on to SDS-PAGE, but the fibrinogenolytic activities have not been quantified. In the present study, we have estimated the fibrinogenolytic activity to calculate the ratio of fibrinolytic to fibrinogeolytic activity, which in turn provides information about the specificity of the enzyme towards fibrin. Eight isolates obtained upon plating the sea water collected from a depth of 10 m, 5 km away from Surathkal Beach in the Arabian Sea were subjected to initial qualitative screening studies using fibrin agar screening medium. Of all the isolates, only six isolates showed the appearance of turbid zone initially, indicating the conversion of fibrinogen to fibrin due to fibrinogenolytic activity, followed by the subsequent appearance of a clear zone within the turbid zone indicating the fibrinolytic activity. Estimation of fibrinolytic activity and fibrinogenolytic activity of cell free broth obtained from each individual isolates showed that the ratio ranged from 6:1 to 8:1. Similar results were reported in the aqueous extracts of Moringa oleifera (Moringaceae) leaves[24], root and latex extracts of Asclepiadaceae plants25, and Calotropis gigantean17. Here, the increase in absorbance is due to the increase in turbidity as a result of fibrinogenolytic activity involving the conversion of fibrinogen to fibrin clot. After reaching the peak absorbance, a gradual decrease was observed in all the cases, perhaps due to the breakdown of the fibrin clot into the fibrin degradation products.
Further, anticoagulant and thrombolytic effect of each culture was assessed by carrying out in vitro assays using human blood and cell free broth. The in vitro studies conducted revealed that the isolate C7 exhibited significant anticoagulant and thrombolytic activities, in comparison to the other five isolates. Similar results were obtained by Ju et al.26 and Lu et al.19 with the bacterial strains Streptomyces sp. XZNUM 00004 and Paenibacillus polymyxa EJS-3 respectively. The results obtained, also concur with the findings of Simkhada et al.[27]. They quoted that the fibrinolytic enzyme FP28, produced by a novel Streptomyces strain showed significant thrombolytic activity, in comparison to the saline and plasmin treated samples, wherein, the dispersed colour density of the sample treated with FP28, when measured spectrophotometrically (405 nm), was higher than that in the plasmin treated sample. Ratio of fibrinolytic to fibrinogenolytic activity, in vitro thrombolytic and anticoagulant activities, fibrinolytic activity upon partial purification, and enzyme activity at physiological range of pH of the isolates were determined. The isolate C7 showed superior performance in every aspect and hence was chosen for further studies.
16S rRNA gene sequencing revealed that the chosen marine organism C7 is Serratia marcescens subsp. sakuensis. Serratia marcescens is a gram-negative bacterium, facultative anaerobe belonging to the family Enterobacteriaceae. It is a widely distributed saprophytic bacterium[28]. Serratia marcescens has long been studied for the production of enzyme Serratiopeptidase, one of the most widely used nonsteroidal anti-inflammatory drugs. The enzyme Serratiopeptidase, is a multifunctional enzyme with potent fibrinolytic activity in addition to its anti-inflammatory, caesinolytic and gelatinolytic properties and is primarily administered as a nonsteroidal anti-inflammatory drug[29]. In view of unavailability of reports exploiting the fibrinolytic activity of proteolytic enzymes of Serratia spp., particularly marine Serratia marcescens subsp. sakuensis, the present work becomes novel. Medium optimisation experiments using Plackett-Burman design and subsequent one factor experiments showed that the chosen organism (Serratia marcescens subsp. sakuensis) has a high requirement for nitrogen and the presence or absence of a carbon source in the medium has minimal effect on the fibrinolytic enzyme production. This could be explained by the fact that marine microbial communities utilise the sinking organic carbon as an energy source, because often such microbes are found in nitrogen limiting conditions, hence they prefer a nitrogen rich environment for their growth[30]. Deepak et al.[31] showed that only peptone had a significant effect on nattokinase production as compared to the other medium components. Likewise, Liu et al.[32] had reported that nitrogen sources such as soya peptone and yeast extract had a significant effect on nattokinase production. Our results were consistent with the reported literature.
In conclusion, seawater could be an excellent source for novel marine microorganisms capable of producing fibrinolytic enzymes. Through a well-designed screening strategy, we could quickly zeroed in on an isolate which was producing fibrinolytic enzyme with desirable attributes such as high specific fibrinolytic activity, higher ratio of fibrinolytic to fibrinogenolytic activity, activity at the physiological pH range, significant anticoagulant and thrombolytic effects in vitro. Using statistical tools in combination with conventional strategies, medium was optimised. The optimised medium gave an enzyme production of 487.29 U/mL, which was a 2.5 fold increase compared to unoptimised medium. Further, enzyme purification, characterization, and in vivo studies are being carried out to ascertain its immunogenicity and effectiveness as a therapeutic drug.
ACKNOWLEDGMENTS
Ms. Anusha Krishnamurthy is supported by scholarship for doctoral course by the National Institute of Technology Karnataka (NITK) Surathkal, Karnataka, India. We thank the volunteers for providing blood for the in vitro experiments.
- Bhardwaj, S., Angayarkanni, J. Streptokinase production from Streptococcus dysgalactiae subsp. equisimilis SK-6 in the presence of surfactants, growth factors and trace elements. 3 Biotech, 2015; (2): 187–193.
- Kotb, E. Activity assessment of microbial fibrinolytic enzymes. Applied Microbiology and Biotechnology, 2013; 97(15): 6647-6665.
- Holden, R. W. Plasminogen activators: pharmacology and therapy. Radiology, 1990; 174: 993-1001.
- Blann, A. D., Landray, M. J., Lip, G. Y. An overview of antithrombotic therapy. British Medical Journal, 2002; 325(7367): 762-765.
- Turpie, A. G., Chin, B. S., Lip, G. Y. Venous thromboembolism: treatment strategies. British Medical Journal, 2002; 325: 945-950.
- Moon, S. M., Kim, J. S., Kim, H. J., Choi, M. S., Park, B. R., Kim, S. G., Ahn, H., Chun, H. S., Shin, Y. K., Kim, J. J., Kim, D. K., Lee, S. Y., Seo, Y. W., Kim, Y. H., Kim, C. S. Purification and characterisation of a novel fibrinolytic á chymotrypsin like serine metalloprotease from the edible mushroom, Lyophyllum shimeji. Journal of Bioscience and Bioengineering, 2014; 117(5): 544-550.
- Prihanto, A. A., Darius, Firdaus, M. Proteolytic and Fibrinolytic Activities of Halophilic Lactic Acid Bacteria From Two Indonesian Fermented Foods. Journal of Microbiology, Biotechnology and Food Sciences, 2013; 2(5): 2291-2293.
- Chitte, R. R., Dey, S. Potent fibrinolytic enzyme from a thermophilic Streptomyces megasporus strain SD5. Letters in Applied Microbiology, 2000; 31(6): 405-410.
- Solingen, P., Dean, V. M., Wilhelmus, A. H. K., Chirstopher, B., Robertus, B., Scott, D. P., Brian, E. J. A novel Streptomyces isolated from an East African Soda Lake. Extremophiles, 2001; 5(5): 333-341.
- Mayer, A., Hamann, M. Marine Pharmacology in 2000: Marine Compounds Antituberculosis, and Antiviral Activities; Affecting the Cardiovascular, Immune, and Nervous Systems and other Miscellaneous Mechanisms of Action. Marine Biotechnology, 2004; 6(1): 37-52.
- Agrebi, R., Haddar, A., Hmidet, N., Jellouli, K., Manni, L., Nasri, M. BSF1 fibrinolytic enzyme from a marine bacterium Bacillus subtilis A26: Purification, biochemical and molecular characterisation. Process Biochemistry, 2009; 44(11): 1252-1259.
- Mahajan, P., Nayak, S., Lele, S. Fibrinolytic enzyme from newly isolated marine bacterium Bacillus subtilis ICTF-1: Media optimisation, purification and characterisation. Journal of Bioscience and Bioengineering, 2012; 113(3): 307-314.
- Huang, S., Pan, S., Chen, G., Huang, S., Zhang, Z., Li, Y., Liang, Z. Biochemical characteristics of a fibrinolytic enzyme purified from a marine bacterium, Bacillus subtilis HQS-3. International Journal of Biological Macromolecules, 2013; 62: 124-130.
- Sharkova, T., Kurakov, A., Osmolovskiy, A., Matveeva, E. O., Kreyer, V. G., Baranova, N. A., Egorov, N. S. Screening of producers of proteinases with fibrinolytic and collagenolytic activities among Micromycetes. Microbiology, 2015; 84(3): 359-364.
- Das, S., Lyla, P. S., Khan, S. A. Marine microbial diversity and ecology: importance and future perspectives. Current Science, 2006; 90(10): 1325-1335.
- Astrup, T., Mullertz, S. The fibrin plate method of estimating of fibrinolytic activity. Archives of Biochemistry and Biophysics, 1952; 40(2): 346-351.
- Shivaprasad, H. V., Rajesh, R., Nanda, B. L., Dharmappa, K. K., Vishwanath, B. S. Cysteine proteases from the Asclepiadaceae plants latex exhibited thrombin and plasmin like activities. Journal of Thrombosis and Thrombolysis, 2009; 28(3): 304-308.
- Wang, S. L., Chen, H. J., Liang, T. W., Lin, Y. D. A novel nattokinase produced by Pseudomonas sp. TKU015 using shrimp shells as substrate. Process Biochemistry, 2009; 44(1): 70-76.
- Lu, F., Lu, Z., Bie, X., Yao, Z., Wang, Y., Lu, Y., Guo, Y. Purification and characterisation of a novel anticoagulant and fibrinolytic enzyme produced by endophytic bacterium Paenibacillus polymyxa EJS-3. Thrombosis Research, 2010; 126(5): 349-355.
- Lowry, O. H., Rosebrough, N. J., Farr, A. L., Randall, R. J. Protein measurement with the Folin phenol reagent. Journal of Biological Chemistry, 1951; 193(1): 265–275.
- Ajithkumar, B., Ajithkumar, V., Iriye, R., Doi, Y., Sakai, T. Spore-forming Serratia marcescens subsp. sakuensis subsp. nov., isolated from a domestic wastewater treatment tank. International Journal of Systematic and Evolutionary Microbiology, 2003; 53: 253-258.
- Mander, P., Cho, S. S., Simkhada, J. R., Choi, Y. H., Yoo, J. C. A low molecular weight chymotrypsin-like novel fibrinolytic enzyme from Streptomyces sp. CS624. Process Biochemistry, 2011; 46(7): 1449-1455.
- Yogesh, D., Halami, P. M. A fibrin degrading serine metalloprotease of Bacillus circulans with á-chain specificity. Food Bioscience, 2015; 11(1): 72-78.
- Satish, A., Sairam, S., Ahmed, F., Urooj, A. Moringa oleifera Lam.: Protease activity against blood coagulation cascade. Pharmacognosy Research, 2012; 4(1): 44-49.
- Bindhu, O. S., Singh, M. K. Hemostatic, milk clotting and blood stain removal potential of cysteine proteases from Calotropis gigantea (L.) R. Br. Latex. Pharmacognosy Magazine, 2014; 10: 350-356.
- Ju, X., Cao, X., Sun, Y., Wang, Z., Cao, C., Liu, J., Jiang, J. Purification and characterisation of a fibrinolytic enzyme from Streptomyces sp. XZNUM 00004. World Journal of Microbiology and Biotechnology, 2012; 28(7): 2479-2486.
- Simkhada, J. R., Cho, S. S., Mander, P., Choi, Y. H., Yoo, J. C. Purification, biochemical properties and antithrombotic effect of a novel Streptomyces enzyme on carrageenan-induced mice tail thrombosis model. Thrombosis Research, 2012; 129(2): 176-182.
- Hejazi, A., Falkiner, F. Serratia marcescens. Journal of Medical Microbiology, 1997; 46(11): 903-912.
- Bhagat, S., Agarwal, M., Roy, V. Serratiopeptidase: A systematic review of the existing evidence. International Journal of Surgery, 2013; 11(3): 209-217.
- Emerson, S., Hedges, J.: Sediment diagenesis and benthic flux. In: Treatise on Geochemistry (Holland HD, Turekian KK, ed). Amsterdam: Elsevier, 2003.
- Deepak, V., Kalishwaralal, K., Ramkumarpandian, S., Babu, S. V., Senthilkumar, S. R., Sangiliyandi, G. Optimisation of media composition for Nattokinase production by Bacillus subtilis using response surface methodology. Bioresource Technology, 2008; 99(17): 8170-8174.
- Liu, J. G., Xing, J. M., Chang, T. S., Ma, Z. Y., Liu, H. Z. Optimisation of nutritional conditions for nattokinase production by Bacillus natto NLSSE using statistical experimental methods. Process Biochemistry, 2005; 40(8): 2757-2762.
© The Author(s) 2017. Open Access. This article is distributed under the terms of the Creative Commons Attribution 4.0 International License which permits unrestricted use, sharing, distribution, and reproduction in any medium, provided you give appropriate credit to the original author(s) and the source, provide a link to the Creative Commons license, and indicate if changes were made.